
First Atomic Freeze-Frame Captures Electrons in Liquid Water
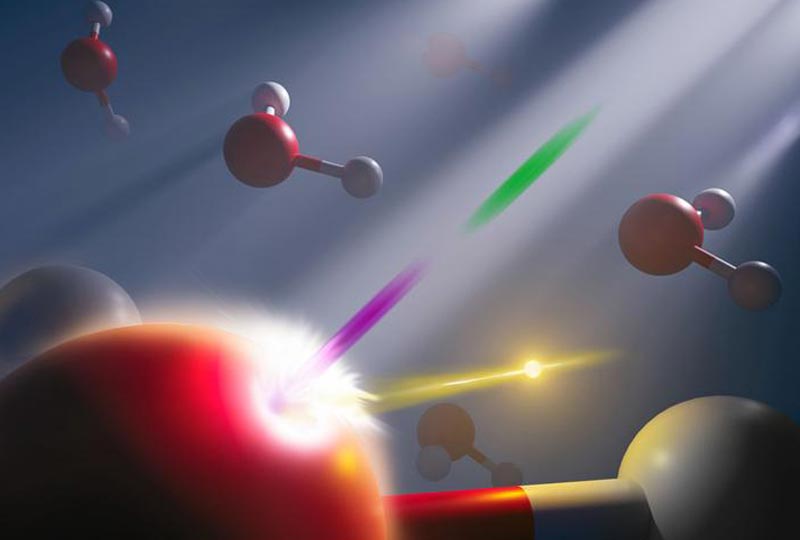
Scientists used a synchronized attosecond X-ray pulse pair (pictured pink and green here) from an X-ray free electron laser to study the energetic response of electrons (gold) in liquid water on attosecond time scale, while the hydrogen (white) and oxygen (red) atoms are ‘frozen’ in time.
Credit: Nathan Johnson | Pacific Northwest National Laboratory
Scientists report the first look at electrons moving in real-time in liquid water; findings open up a whole new field of experimental physics.
In an experiment akin to stop-motion photography, scientists have isolated the energetic movement of an electron while “freezing” the motion of the much larger atom it orbits in a sample of liquid water.
The findings, reported today in the journal Science, provide a new window into the electronic structure of molecules in the liquid phase on a timescale previously unattainable with X-rays. The new technique reveals the immediate electronic response when a target is hit with an X-ray, an important step in understanding the effects of radiation exposure on objects and people.
“The chemical reactions induced by radiation that we want to study are the result of the electronic response of the target that happens on the attosecond timescale,” said Linda Young, a senior author of the research and Distinguished Fellow at Argonne National Laboratory. “Until now radiation chemists could only resolve events at the picosecond timescale, a million times slower than an attosecond. It’s kind of like saying ‘I was born and then I died.’ You’d like to know what happens in between. That’s what we are now able to do.”
A multi-institutional group of scientists from several Department of Energy national laboratories and universities in the U.S. and Germany combined experiments and theory to reveal in real-time the consequences when ionizing radiation from an X-ray source hits matter.
Working on the time scales where the action happens will allow the research team to understand complex radiation-induced chemistry more deeply. Indeed, these researchers initially came together to develop the tools needed to understand the effect of prolonged exposure to ionizing radiation on the chemicals found in nuclear waste. The research is supported by the Interfacial Dynamics in Radioactive Environments and Materials (IDREAM) Energy Frontier Research Center sponsored by the Department of Energy and headquartered at Pacific Northwest National Laboratory (PNNL).
“Members of our early-career network participated in the experiment, and then joined our full experimental and theoretical teams to analyze and understand the data,” said Carolyn Pearce, IDREAM EFRC director and a PNNL chemist. “We couldn’t have done this without the IDREAM partnerships.”
From the Nobel Prize to the field
Subatomic particles move so fast that capturing their actions requires a probe capable of measuring time in attoseconds, a time frame so small that there are more attoseconds in a second than there have been seconds in the history of the universe.
The current investigation builds upon the new science of attosecond physics, recognized with the 2023 Nobel Prize in Physics. Attosecond X-ray pulses are only available in a handful of specialized facilities worldwide. This research team conducted their experimental work at the Linac Coherent Light Source (LCLS), located at SLAC National Accelerator Laboratory, in Menlo Park, Calif, where the local team pioneered the development of attosecond X-ray free-electron lasers.
“Attosecond time-resolved experiments are one of the flagship R&D developments at the Linac Coherent Light Source,” said Ago Marinelli from the SLAC National Accelerator Laboratory, who, together with James Cryan, led the development of the synchronized pair of X-ray attosecond pump/probe pulses that this experiment used. “It’s exciting to see these developments being applied to new kinds of experiments and taking attosecond science into new directions.”
The technique developed in this study, all X-ray attosecond transient absorption spectroscopy in liquids, allowed them to “watch” electrons energized by X-rays as they move into an excited state, all before the bulkier atomic nucleus has time to move. They chose the liquid water as their test case for an experiment.
“We now have a tool where, in principle, you can follow the movement of electrons and see newly ionized molecules as they’re formed in real-time,” said Young, who is also a professor in the Department of Physics and James Franck Institute at the University of Chicago.
These newly reported findings resolve a long-standing scientific debate about whether X-ray signals seen in previous experiments are the result of different structural shapes, or “motifs,” of water or hydrogen atom dynamics. These experiments demonstrate conclusively that those signals are not evidence for two structural motifs in ambient liquid water.
“Basically, what people were seeing in previous experiments was the blur caused by moving hydrogen atoms,” said Young. “We were able to eliminate that movement by doing all of our recording before the atoms had time to move.”
From simple to complex reactions
The researchers envision the current study as the beginning of a whole new direction for attosecond science.
To make the discovery, PNNL experimental chemists teamed with physicists at Argonne and the University of Chicago, X-ray spectroscopy specialists and accelerator physicists at SLAC, theoretical chemists at the University of Washington, and attosecond science theoreticians from the Hamburg Centre for Ultrafast Imaging and the Center for Free-Electron Laser Science (CFEL), Deutsches Elektronen-Synchrotron (DESY), in Hamburg, Germany.
During the global pandemic, in 2021 and into 2022, the PNNL team used techniques developed at SLAC to spray an ultra-thin sheet of pure water across the X-ray pump pulse path.
“We needed a nice, flat, thin sheet of water where we could focus the X-rays,” said Emily Nienhuis, an early-career chemist at PNNL, who started the project as a post-doctoral research associate. “This capability was developed at the LCLS.” At PNNL, Nienhuis demonstrated that this technique can also be used to study the specific concentrated solutions that are central to the IDREAM EFRC and will be investigated at the next stage of the research.
From experiment to theory
Once the X-ray data had been collected, theoretical chemist Xiaosong Li and graduate student Lixin Lu from the University of Washington applied their knowledge of interpreting the X-ray signals to reproduce the signals observed at SLAC. The CFEL team, led by theoretician Robin Santra, modelled the liquid water response to attosecond X-rays to verify that the observed signal was indeed confined to the attosecond timescale.
“Using the Hyak supercomputer at the University of Washington, we developed a cutting-edge computational chemistry technique that enabled detailed characterization of the transient high-energy quantum states in water,” said Li, the Larry R. Dalton Endowed Chair in Chemistry at the University of Washington and a Laboratory Fellow at PNNL. “This methodological breakthrough yielded a pivotal advancement in the quantum-level understanding of ultrafast chemical transformation, with exceptional accuracy and atomic-level detail.”
Principal Investigator Young originated the study and supervised its execution, which was led on-site by first author and postdoc Shuai Li. Physicist Gilles Doumy, also of Argonne, and graduate student Kai Li of the University of Chicago were part of the team that conducted the experiments and analyzed the data. Argonne’s Center for Nanoscale Materials, a DOE Office of Science user facility, helped characterize the water sheet jet target.
Together, the research team got a peek at the real-time motion of electrons in liquid water while the rest of the world stood still.
“The methodology we developed permits the study of the origin and evolution of reactive species produced by radiation-induced processes, such as encountered in space travel, cancer treatments, nuclear reactors and legacy waste,” said Young.
The study has three co-first authors: S. Li, Lu, and Swarnendu Bhattacharyya of DESY. The three corresponding authors are X. Li, Santra and Young. A full author list is available here.
This work was primarily supported by IDREAM, an Energy Frontier Research Center funded by the Department of Energy, Office of Science, Basic Energy Sciences program. Use of the LCLS, the SLAC National Accelerator Laboratory, and resources from the Center for Nanoscale Materials, Argonne National Laboratory, are supported by the DOE Office of Science, Basic Energy Sciences program. Additional support came from DESY and Cluster of Excellence, “CUI: Advanced Imaging of Matter,” of the Deutsche Forschungsgemeinschaft.
About PNNL
Pacific Northwest National Laboratory draws on its distinguishing strengths in chemistry, Earth sciences, biology and data science to advance scientific knowledge and address challenges in sustainable energy and national security. Founded in 1965, PNNL is operated by Battelle for the Department of Energy’s Office of Science, which is the single largest supporter of basic research in the physical sciences in the United States. DOE’s Office of Science is working to address some of the most pressing challenges of our time. For more information, visit https://energy.gov/science. For more information on PNNL, visit PNNL’s News Center. Follow us on Twitter, Facebook, LinkedIn and Instagram.
Journal: Science
DOI: 10.1126/science.adn6059
Method of Research: Experimental study
Subject of Research: Not applicable
Article Title: Attosecond-pump attosecond-probe x-ray spectroscopy of liquid water
Article Publication Date: 15-Feb-2024
Media Contact
Karyn Hede
DOE/Pacific Northwest National Laboratory
karyn.hede@pnnl.gov
Office: 509-375-2144